On 1 April 2019 LIGO and Virgo began their third observing run (O3). Never before had we observed using such sensitive gravitational wave detectors. Throughout O3 discoveries came rapidly. Binary black holes are our most common source, and as we built a larger collection we starting to find some unusual systems. GW190412 is our first observation from a binary with two distinctly differently sized black holes. This observation lets us test our predictions for gravitational wave signals in a new way, and is another piece in the puzzle of understanding how binary black holes form.
The discovery
On 12 April 2019 I awoke to the news that we had a new gravitational wave candidate [bonus note]. The event was picked up by our searches and sent out as a public alert under the name S190412m. The signal is a real beauty. There’s a striking chirp visible in the Livingston data, and a respectable chirp in the Hanford data. You can’t see a chirp in Virgo, the signal-to-noise ratio is only about 4, but this is why we have cunning search algorithms instead of looking at the data by eye. In our final search results, our matched-filter searches (which use templates of gravitational wave signals to comb through the data) GstLAL and PyCBC identified the event with false alarm rates of better than 1 in 100,000 years and 1 in 30,000 years, respectively. Our unmodelled search coherent WaveBurst (which looks for compatible signals in multiple detectors, rather than a specific template) also identified the event with a false alarm rate of better than 1 in 1,000 years. This is a confident detection!
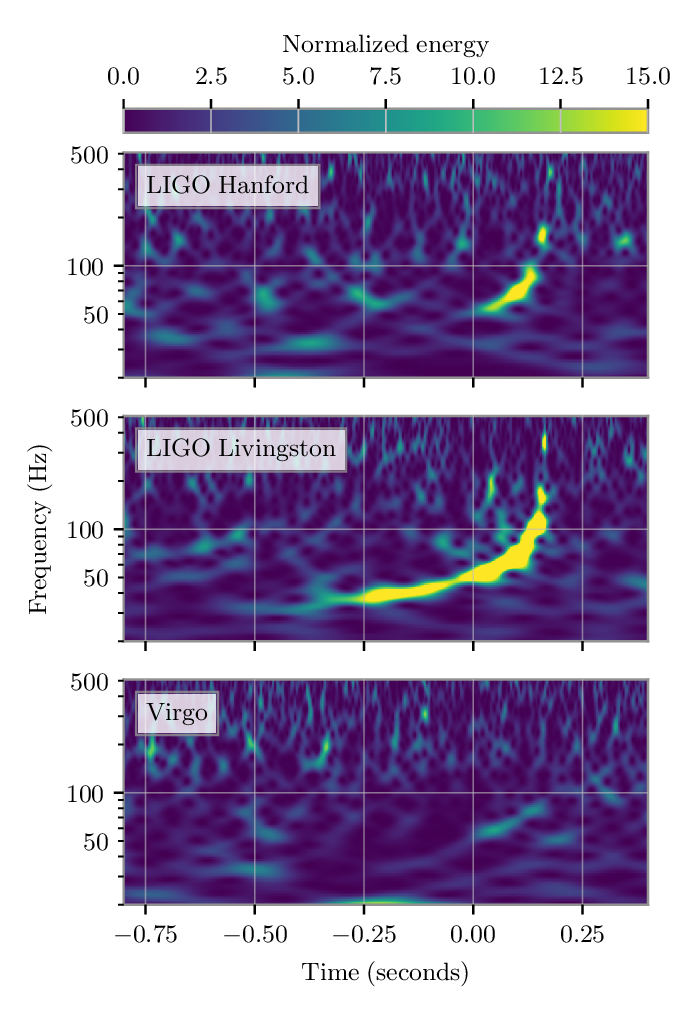
Time–frequency plots for GW190412 as measured by LIGO Hanford, LIGO Livingston and Virgo. The chirp of a binary coalescence is clearer in two LIGO detectors, with the signal being loudest in Livingston. Figure 1 of the GW190412 Discovery Paper.
Vanilla black holes
Our first gravitational wave detection, GW150914, was amazing. We had never seen a black hole around 30 times the mass of our Sun, and here we had two merging together (which we had also never seen). By the end of our second observing run, we had discovered that GW150914 was not rare! Many of detections consisted of two roughly equal mass black holes around 20 to 40 times the mass of Sun. We now call these systems vanilla binary black holes. They are nice and easy to analyse: we know what to do, and it’s not too difficult. I think that these signals are delicious.
GW190412’s source, however, is different. We estimate that the binary had one black hole times the mass of our Sun (quoting the 90% range for parameters), and the other
times the mass of our Sun. Neither of these masses is too surprising on their own. We know black holes come in these sizes. What is new is the ratio of the masses
[bonus note]. This is roughly equal to the ratio of filling in a regular Oreo to in a Mega Stuf Oreo. Investigations of connections between Oreos and black hole formation are ongoing. All our previous observations have mass ratios close to 1 or at least with uncertainties stretching all the way to 1. GW190412’s mass ratio is the exception.
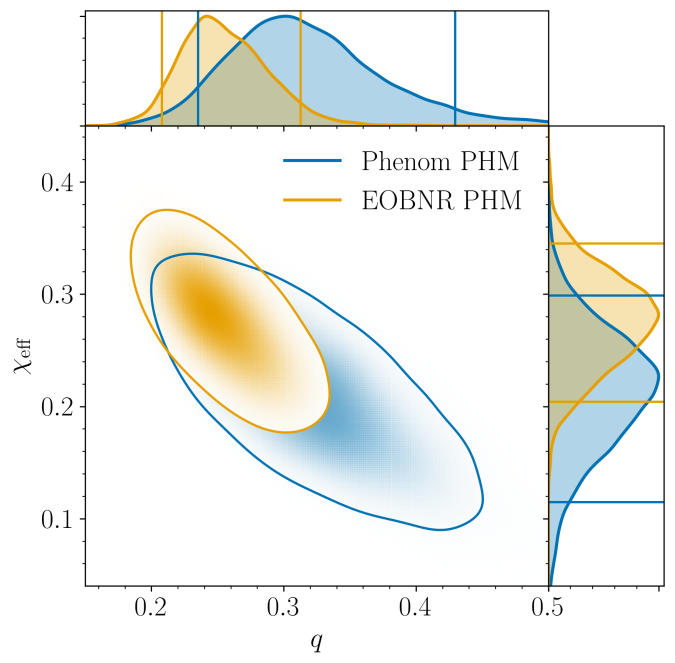
Estimated mass ratio for the two components in the binary and the effective inspiral spin
(a mass-weighted combination of the spins perpendicular to the orbital plane). We show results for two different model waveforms: Phenom PHM and EOB PHM (the PHM stands for precession and higher order multipoles). Systems with unequal masses are difficult to model, so we have some extra uncertainty from the accuracy of our models. The two-dimensional shows the 90% probability contour. The one-dimensional plots show the probability distributions and the the dotted lines mark the central 90%. Figure 2 of the GW190412 Discovery Paper.
The interesting mass ratio has a few awesome implications:
- We get a really wonderful measurement of the spin of the more massive black hole.
- We can observe a new feature of the gravitational wave signal (higher order multipole moments).
- We understand a bit more about the population of binary black holes.
Spin
Black holes have two important properties: mass (how much the bend spacetime) and spin (how much they swirl spacetime around). The black hole masses are most important for determining what a gravitational wave signal looks like, so we measure the masses pretty well. Spins leave a more subtle imprint, and so are more difficult to measure.
A well measured, and convenient to work with, combination of the two spins is the effective inspiral spin parameter
,
where and
are the spins of the two black holes [bonus note], and
and
are the tilts angles measuring the alignment of the spins with the orbital angular momentum. The spins change orientations during the inspiral if they are not perfectly aligned with the orbital angular momentum, which is referred to as precession, but
is roughly constant. It also effects the rate of inspiral, binaries with larger
also merge when they’re a bit closer. For GW190412, we measure
.
This is only the second time we’ve had a definite non-zero measurement of after GW151226. GW170729 had a reasonable large value, but the uncertainties did stretch to include zero. The measurement of a non-zero
means that we know at least one of the black holes has spin.
The effective inspiral spin parameter measures the spin components aligned with the orbital angular momentum. To measure the spin components in the orbital plane, we typically use the effective precession spin parameter [bonus note]
.
This characterises how much spin precession we have: 1 means significant in-plane spin and maximal precession, and zero means no in-plane spin and no precession.
For GW190412, we measure . This is the best measurement of
so far. It shows that we don’t see strong procession, but also suggests that there is some in-plane spin.
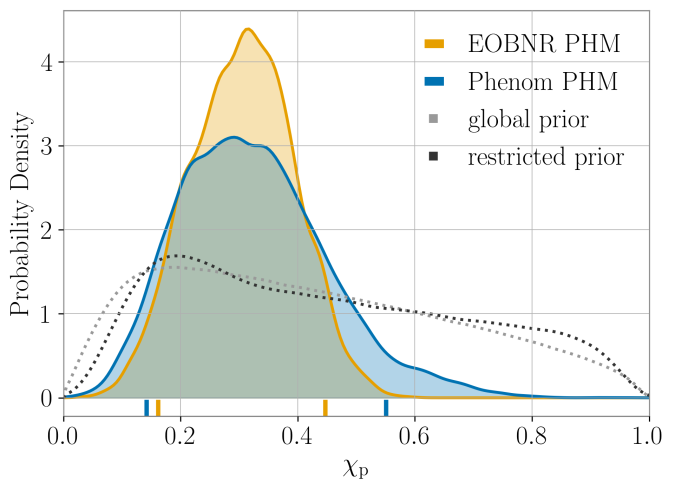
Estimated effective precession spin parameter . Results are shown for two different waveform models. To indicate how much (or little) we’ve learnt, the prior probability distribution is shown: the global prior is what we would get if we had learnt nothing, the restricted prior is what we would have after placing cuts on the effective inspiral spin parameter and mass ratio to match our observations. We are definitely getting information on precession from the data. Figure 5 of the GW190412 Discovery Paper.
Now, since we know that the masses are unequal in the binary, the contribution to is dominated by the spin of the larger black hole, or at least the component of the spin aligned with the orbital angular momentum (
), and similarly
is dominated by the in-place components of the larger black hole’s spin (
). Combining all this information, we can actually get a good measurement of the spin of the bigger black hole. We infer that
. This is the first time we’ve really been able to measure an individual spin!
We don’t yet have a really good understanding of the spins black holes are born with. Their spins can increase if they accrete material, but it needs to be a lot of stuff to change it significantly. When we make a few more spin measurements, I’m looking forward to using the information to help figure out the histories of our black holes.
Higher order multipoles
When calculating gravitational wave signals, we often use spin-weighted spherical harmonics. These are a set of functions, which describe possible patterns on a sphere. Using them, we can describe the amount of gravitational waves emitted in a particular direction. Any gravitational wave signal can be approximated as a sum of the spin-weighted spherical harmonics , where we use
as the angles on the sphere, and
specify the harmonic. The majority of the gravitational radiation emitted from a binary is from the
harmonic, so we usually start with this. Larger values of
contribute less and less. For exactly equal mass binaries with non-spinning components, only harmonics with even
are non-zero, so really the
harmonic is all you need. For unequal mass binaries this is not the case. Here odd
are important, and harmonics with
are expected to contribute a significant amount. In previous detection, we’ve not had to worry too much about the harmonics with
, which we refer to as higher order multipole moments, as they contributed little to the signal. GW190412’s unequal masses mean that they are important here.
During the inspiral, the frequency of the part of the gravitational wave signal corresponding to a given is
, where
is the orbital frequency. Most of the signal is emitted at twice the orbital frequency, but the emission from the higher order multipoles is at higher frequencies. If the
multipole was a music A, then the
multipole would correspond to an E, and if the
multipole was a C, the
would be a G. There’s a family of chirps [bonus note]. For GW190412, we clearly pick out the frequency component at
showing the significance of the
mode. This shows that the harmonic structure of gravitational waves is as expected [bonus note]. We have observed a perfect fifth, as played by the inspiral of two black holes.
Using waveforms which include higher order multipoles is important to get good measurements of the source’s parameters. We would not get a good measurement of the mass ratio or the distance (, corresponding to a travel time for the signal of around 2 billion years) using templates calculated using only the
harmonic.
The black hole population
GW190412’s source has two unequal mass black holes, unlike our vanilla binary black holes. Does this indicate a new flavour of binary black hole, and what can we learn about how it formed from it’s properties?
After our second observing run, we analysed our family of ten binary black holes to infer what the population looked like. This included fitting for the distribution of mass mass ratios. We assumed that the mass ratios were drawn from a distribution something like and estimated the value of
. A result of
would mean that all mass ratios were equally common, while larger values would mean that binaries liked more equal mass binaries. Our analysis preferred larger values of
, making it appear that black holes were picky about their partners. However, with only ten systems, our uncertainties spanned the entire range we’d allowed for
. It was too early to say anything definite about the mass ratio distribution.
If we add in GW190412 to the previous ten observations, we get a much tighter measurement of , and generally prefer values towards the lower end of what we found previously. Really, we shouldn’t just add in GW190412 when making statements about the entire population, we should fold in everything we saw in our observing run. We’re working on that. For now, consider these as preliminary results which would be similar to those we would have got if the observing run was only a couple of weeks long.
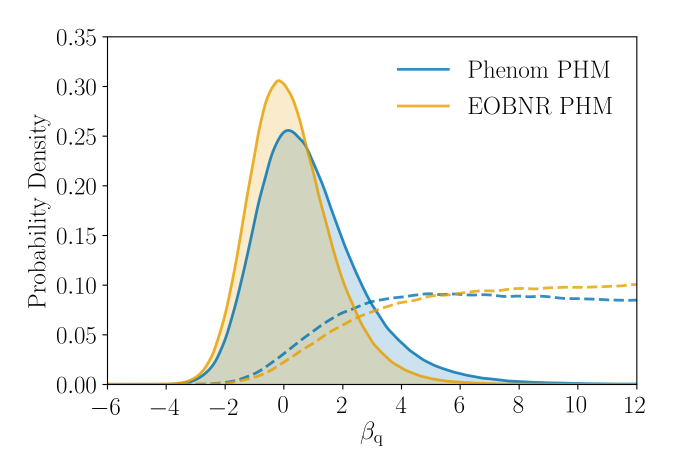
Estimated power-law slope for the binary black hole mass ratio distribution
. Dotted lines show the results with our first ten detections, and solid lines include GW190412. Results are shown for two different waveform models. Figure 11 of the GW190412 Discovery Paper.
Since most of the other binaries are more equal mass, we can see the effects of folding this information into our analysis of GW190412. Instead of making weak assumptions about what we expect the masses to be (we normally assume uniform prior probability on the masses as redshifted and measured in the detector, as that’s easy to work with), we can use our knowledge of the population. In this case, our prior expectation that we should have something near equal mass does shift the result a little, the 90% upper limit for the mass ratio shifts from to
, but we see that the mass ratio is still clearly unequal.
Have we detected a new flavour of binary black hole? Should we be lumping in GW190412 with the others, or should it be it’s own category? Going back to our results from the second observing run, we find that we’d expect that in a set of eleven observations that at least one would have a mass ratio as extreme as GW190412 of the time. Therefore, GW190412 is exceptional, but not completely inconsistent with our previous observations. If we repeat the calculation using the population inferred folding in GW190412, we (unsurprisingly) find it is much less unusual, with such systems being found in a set of eleven observations
of the time. In conclusion, GW190412 is not vanilla, but is possibly raspberry ripple or Neapolitan: there’s still a trace of vanilla in there to connect it to the more usual binaries
Now we’ve compared GW190412 to our previous observations, where does its source fit in with predictions? The two main options for making a merging binary black hole are via isolated evolution, where two stars live their lives together, and dynamical formation, where you have lots of black holes in a dense environment like a globular cluster and two get close enough together to capture each other. Both of these favour more equal mass binaries, with unequal mass binaries like GW190412’s source being rare (but not impossible). Since we’ve only seen one system with such a mass ratio in amongst our detections so far, either channel could possibly explain things. My money is on a mixture.
In case you were curious, calculations from Chase Kimball indicate that GW190412 is not a hierarchical merger with the primary black hole being formed from the merger of two smaller black holes.
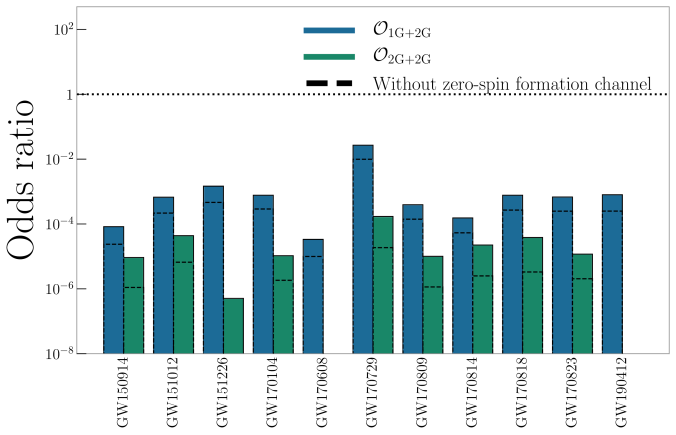
Odds of binary black holes being a hierarchical merger verses being original generation binary. 1G indicates first generation black holes formed from the collapse of stars, 2G indicates a black hole formed from the merger of two 1G black holes. These are preliminary results using the GWTC-1 results plus GW!90412. Fig. 15 of Kimball et al. (2020).
As we build up a larger collection of detections, we’ll be able to use our constraints on the population to better understand the relative contributions from the different formation mechanisms, and hence the physics of black hole manufacturing.
Einstein is not wrong yet
Finally, since GW190412 is beautifully loud and has a respectably long inspiral, we were able to perform our usual tests of general relativity and confirm that all is as predicted.
We performed the inspiral/merger–ringdown consistency test, where we check that parameters inferred from the early, low frequency part of the signal match those from the later, high frequency part. They do.
We also performed the parameterized test, where we we allow different pieces of the signal template vary. We found that all the deviations were consistent with zero, as expected. The results are amongst the tightest we have from a single event, being comparable to results from GW151226 and GW170608. These are the lowest mass binary black holes we’ve observed so far, and so have the longest chirps.
We’ll keep checking for any evidence that Einstein’s theory of gravity is wrong. If Columbo has taught us anything, it is that the guest star is usually guilty. If it’s taught us something else, it’s the importance of a good raincoat. After that, however, it’s taught us the importance of perseverance, and always asking one more thing. Maybe we’ll catch Einstein out eventually.
Just a taste of what’s to come
GW190412 was observed on the 12th day of O3. There were many detections to follow. Using this data set, we’ll be able to understand the properties of black holes and gravitational waves better than ever before. There are exciting results still being finalised.
Perhaps there will be a salted caramel binary black hole, or even a rocky road flavoured one? We might need to wait for our next observing run in 2021 for sprinkles though.
Title: GW190412: Observation of a binary-black-hole coalescence with asymmetric masses
Journal: Physical Review D; 102(4):043015(29)
arXiv: 2004.08342 [astro-ph.HE]
Science summary: GW190412: The first observation of an unequal-mass black hole merger
Data release: Gravitational Wave Open Science Center
Rating: 🍨🐦🎶🐦🥴
Bonus notes
Sleep
I like sleep. I’d strongly recommend it.
Notation
Possibly the greatest dispute in gravitational wave astronomy is the definition of . We pretty much all agree that the larger mass in a binary is
and the lesser mass
. However, there two camps on the mass ratio: those enlightened individuals who define
, meaning that the mass ratio spans the entirely sensible range of
, and those heretics who define
, meaning that it cover the ridiculous range of
. Within LIGO and Virgo, we have now settled on the correct convention. Many lives may have been lost, but I’m sure you’ll agree that it is a sacrifice worth making in the cause of consistent notation.
The second greatest dispute may be what to call the spin magnitudes. In LIGO and Virgo we’ve often used both (the Greek letter chi) and
. After a tense negotiation, conflict was happily avoided, and we have settled on
, with only the minimum amount of bloodshed. If you’re reading some of our older stuff, please bear in mind that we’ve not been consistent about the meaning of these symbols.
Effective spins
Sadly, my suggestions to call and
Chip and Dale have not caught on.
Hey! Listen!
Here are two model waveforms (made by Florian Wicke and Frank Ohme) consistent with the properties of GW190412, but shifted in frequency by a factor of 25 to make them easier to hear:
Can you tell the difference? I prefer the more proper one with harmonics.
Exactly as predicted
The presence of higher order multipole moments, as predicted, could be seen as another win for Einstein’s theory of general relativity. However, we expect the same pattern of emission in any theory, as it’s really set by the geometry of the source. If the frequency were not an integer multiple of the orbital frequency, the gravitational waves would get out of phase with their source, which would not make any sense.
The really cool thing, in my opinion, is that we now how detectors sensitive enough to pick out these subtle details.
Nice Article.!
I have a naive question:
Suppose the f_orb is 2Hz.
And the detector sees a GW of 6Hz.
How can you tell if this is an m=3 wave?
Or the chirping up of an m=2 wave?
LikeLike
This is a good question, and something that we’ve worried about. The (2, 2) multipole moment should always be louder, which means you shouldn’t be able to detect only the higher order multipole moments and not the (2, 2) contribution. As soon as you have seen many harmonics, you can identify which is which by their frequencies: the (2, 2) being lowest. You could miss something if the (2, 2) multipole moment was too low frequency (below our detector’s range), and so you only saw the higher order multipole. That’s unlikely, as there’s only a small range of frequencies where that could happen, and for coalescing binaries sweep through a range of frequencies.
LikeLike